When you experience something with your senses, it evokes complex patterns of activity in your brain. One important goal in neuroscience is to decipher how these neural patterns drive the sensory experience.
For example, can the smell of chocolate be represented by a single brain cell, groups of cells firing all at the same time or cells firing in some precise symphony? The answers to these questions will lead to a broader understanding of how our brains represent the external world. They also have implications for treating disorders where the brain fails in representing the external world: for example, in the loss of sight of smell.
To understand how the brain drives sensory experience, my colleagues and I focus on the sense of smell in mice. We directly control a mouse’s neural activity, generating “synthetic smells” in the olfactory part of its brain in order to learn more about how the sense of smell works.
Our latest experiments discovered that scents are represented by very specific patterns of activity in the brain. Like the notes of a melody, the cells fire in a unique sequence with particular timing to represent the sensation of smelling a unique odor.
Scents produced by light projections
Using mice to study smell is appealing to researchers because the relevant brain circuits have been mapped out, and modern tools allow us to directly manipulate these brain connections.
The mice we use are genetically engineered so we can activate individual brain cells simply by shining light of specific wavelengths onto them – a technique known as optogenetics. Early uses of optogenetics involved light delivered through implanted optical fibers, letting researchers control coarse patches of brain cells. More recent uses of optogenetics allow more sophisticated control of precise patterns of brain activity.
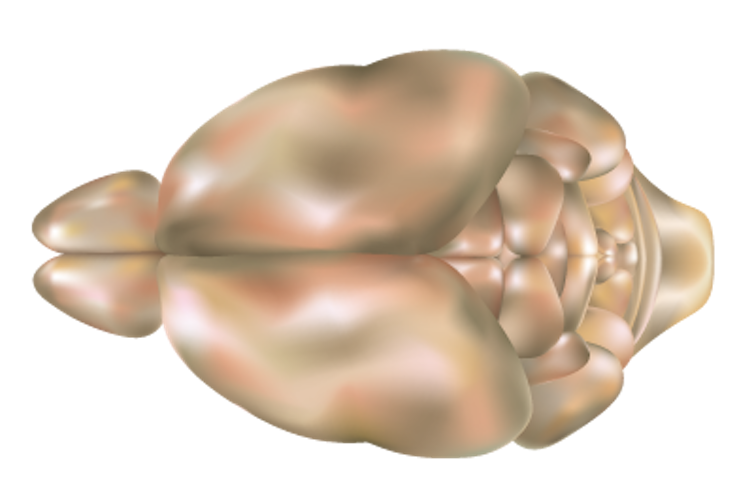
Database Center for Life Science/Wikimedia Commons, CC BY
For our study, we projected light patterns onto the surface of the brain, targeting a region known as the olfactory bulb. Previous research has found that when mice sniff different scents, cells in the olfactory bulb appear to fire in a sort of patterned symphony, with a unique pattern formed in response to each distinct smell.
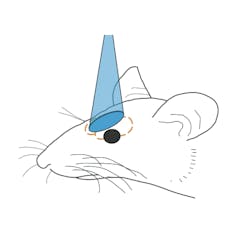
Edmund Chong, CC BY-ND
When we shined light patterns onto a mouse’s olfactory bulb, it generated corresponding patterns of cellular activity. We called these patterns “synthetic smells.” As opposed to a pattern of activity triggered by a mouse sniffing a real odor, we directly triggered the neural activity of a “synthetic smell” with our light projections.
Next we trained each individual mouse to recognize a randomly generated synthetic smell. Since they can’t describe to us in words what they’re perceiving, we rewarded each mouse with water if it licked a water spout whenever it detected its assigned smell. Over weeks of training, mice learned to lick when their assigned smell was activated, and not to lick for other randomly generated synthetic smells.
We cannot say for sure that these synthetic smells correspond to any known odor in the world, nor do we know what they smell like to the mouse. But we did calibrate our synthetic patterns to broadly resemble olfactory bulb patterns observed when actual scents are present. Furthermore, mice learn to discriminate synthetic smells about as quickly as they did real smells.
Tweaking the pattern of a synthetic smell
Once each mouse learned to recognize its assigned synthetic smell, we measured how much they still licked when we modified the assigned smell. Within each synthetic pattern, we altered which cells were activated or when they activated.
Imagine taking a familiar song, changing individual notes in the song, and asking whether you still recognized the song after each change. By testing many different changes, one can eventually understand which precise composition of notes is essential to the song’s identity and which tweaks are extreme enough to make the song unrecognizable.
Likewise, by measuring how mice changed their licking as we modified their projected light patterns, we were able to understand which combinations of cells within the pattern were important for identifying the synthetic smell.
The precise combination of cells activated was crucial. But just as important was when they are activated in an ordered sequence, akin to timed notes in a melody. For example, changing the order of cells in the sequence would render the smell unrecognizable.
It turned out that the cells activated earlier in the sequence were more important for recognition – changing the sequences later in the pattern seemed to have negligible effects.
Changes in recognition were graded, and not drastic: When we changed small parts of the pattern, the smell did not become completely unrecognizable. In fact, the degree to which the smell was recognized was proportional to the degree of change in the pattern. This implies that if I slightly modify the brain activity that represents an orange, you would still smell something similar – maybe recognizing it as citrus, or fruity.
So while the brain has huge capacity to store many different smells in unique timed sequences of cell activity, you can still recognize similar smells by the similarity in their patterns: The smell of coffee is still distinctly recognizable even with a splash of vanilla added to it.
The next step in this research is to bring the synthetic approach to real smells. To do so, we would need to record brain activity in response to a real smell, then reactivate the very same cells using optogenetics. The synthetic re-creation of real objects in the brain is the current focus of research in multiple labs including ours.
Addressing this issue is exciting because it opens up possibilities not just for understanding how the brain works, but also for developing brain implants that may one day restore the loss of sensory experiences.
Edmund Chong, New York University
Edmund Chong, Ph.D. Student in Neuroscience, New York University
This article is republished from The Conversation under a Creative Commons license. Read the original article. https://creativecommons.org/licenses/by-nd/4.0/