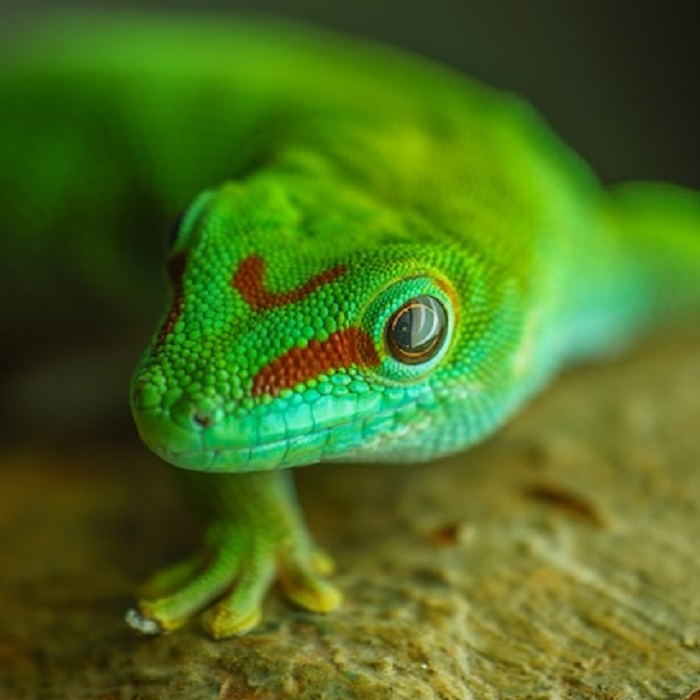
Geckos walk on water – we filmed them to find out how.
Anyone who’s seen a gecko will likely know they can climb walls. But these common lizards can also run across water nearly as fast as they can move on solid ground. Yet while we know how geckos scale smooth vertical surfaces using countless tiny hairs on their feet called setae, how they manage to avoid sinking into the water has been something of a mystery – until now. My colleagues and I recently completed research that explains how geckos use a combination of techniques to perform this amazing feat.
The ability to walk on water has been recorded in smaller animals such as the water strider, which are light enough to be held up by the water’s surface tension, the force between the water molecules at the surface. Meanwhile, larger animals such as the grebe, can walk on water because they are powerful enough to slap the surface with their feet as they run. The fast movement pushes down the water beneath the foot, creating a pocket of air around it. The upwards force generated when this pocket is pushed under the water is what keeps the animal briefly suspended on the surface.
But geckos are typically a size that falls in between these two categories. They are too weak to hold themselves up using surface slapping alone and too heavy to leave the water’s surface unbroken. Yet their relative water running speeds approach those of another well-known water running lizard, the basilisk (or “Jesus lizard”), which does rely on the slapping technique.
Initial calculations hinted, and video analysis confirmed, that unlike other species that move at the water’s surface, geckos use a combination of techniques to move faster on top of the water than they can by swimming through it. By analysing videos of geckos moving across the water, we found that their gait was similar to that of the basilisk. Each step involves retracting the foot through the air, slapping the surface, and stroking beneath the water.
But unlike basilisks, which aren’t affected by changes in the water’s surface tension, our experiments showed that geckos’ speed and head height were cut by half when we added detergent to the water, reducing the surface tension. This suggests that they are at least partly using the forces between the water molecules to stay above the surface.
We also found that geckos crucially use a combination of hydrostatic force (the upwards push of the water known as buoyancy) and hydrodynamic force (the lift created by movement across the water’s surface like in a surface-skimming motorboat). Together, these forces generate additional lift for the gecko, a condition known as semi-planing.
Sting in the tail
For all the ingenuity of this multi-tasking approach, geckos can only keep their head and torso fully above the water, leaving their tails dragging underneath. Being able to move almost as fast as on land when almost half of your body is underwater and facing more resistance and drag forces is quite a feat – just ask Michael Phelps.
Geckos manage this by using their tail, which has already been shown to help them manoeuvre around obstacles, jump and escape predators. Seen from above as it travels across the water, the gecko can resemble a crocodile, moving its body and tail with a wavelike motion to create propulsion to balance the backwards pull of the water.
Our research shows that for medium-sized animals to move quickly along the surface of water a complex and clever combination of physical mechanisms is required that previously was thought only to occur in larger and smaller animals. But it could also feed into better designs for animal-inspired robots.
Previous studies on geckos have inspired several such “biomimetic” endeavours, from better adhesives to an agile (and pretty adorable) tailed robot car, aptly named Tailbot. Better understanding of how animals travel across complex terrains will hopefully lead to robots that can harness these techniques to move on both land and water with the high performance seen in geckos.
Jasmine Nirody, University of Oxford
Jasmine Nirody, Post-Doctoral Research Fellow in Biophysics, University of Oxford
This article is republished from The Conversation under a Creative Commons license. Read the original article.